AVEVA and Petrofac enter MoU to accelerate digital initiatives for the energy industry
Unlock intelligent oil and gas operations with AVEVA software solutions
Decarbonize operations, reduce carbon intensity and ensure compliance
- Gain visibility into carbon performance, set baseline and identify areas of improvement.
- Prevent environmental incidents, manage energy use and minimize waste with real-time monitoring and optimization.
- Design and deliver low-carbon assets and processes through efficient facility design and advanced process simulation.
Learn how TotalEnergies leveraged AVEVA™ PI System™ for energy efficiency and emissions monitoring at scale
Related products
Collect, aggregate and enrich real-time operations data for immediate problem-solving and easily deliver formatted data to enterprise applications and advanced analytics.
Engineer sustainable processes and plants at the speed the market demands. Quantify the impact of potential designs on sustainability, feasibility, and profitability.
Monitor asset health and maximize reliability with purpose-built AI and self-service analytics. Forecast remaining asset life, reduce unplanned downtime and lower maintenance costs.
Capitalize on digital technologies and transform your business by integrating and visualizing all available data.
Streamline capital projects and minimize CapEx with data-centric workflows
- Accelerate engineering cycles for greenfield and brownfield projects.
- Improve visibility and control over capital project execution to deliver facilities on time and on budget, minimize waste and manage stakeholders.
- Leverage digital twins to manage engineering information, drive a continuous handover to operations and increase performance.
Learn how BP and KBR developed a full life-cycle digital twin in offshore Azerbaijan.
Related products
Engineer and collaborate across all project phases and multi-discipline teams in a single data-centric platform.
Engineer sustainable processes and plants at the speed the market demands. Quantify the impact of potential designs on sustainability, feasibility, and profitability.
Accelerate information discovery and break down silos for fast, intuitive, in-context access to relevant data.
Our industrial cloud platform lets you securely access our broad portfolio of intelligence-as-a-service software solutions.
Drive increased asset reliability, performance and safety
- Maximize asset health and performance through real-time monitoring, predictive analytics and advanced alerting.
- Empower field teams with decision-ready data, simplified maintenance workflows and institutionalized knowledge capture.
- Transition from reactive maintenance to a risk-based approach leveraging AI and first-principal simulation.
Learn how Suncor leveraged AVEVA for advanced equipment health monitoring and maintenance planning.
Related products
Collect, aggregate, and enrich real-time operations data for immediate problem-solving and easily deliver formatted data to enterprise applications and advanced analytics.
Engineer sustainable processes and plants at the speed the market demands. Quantify the impact of potential designs on sustainability, feasibility, and profitability.
Monitor asset health and maximize reliability with purpose-built AI and self-service analytics. Forecast remaining asset life, reduce unplanned downtime and lower maintenance costs.
Use real-time and off-line process and economic data to maximize operating profit while satisfying regulatory requirements.
Optimize value chains and increase operational agility
- Accelerate decision-making with data availability and streamlined workflows.
- Leverage simulation and real-time optimization to maximize profitability and sustainability throughout operations.
- Pair live operational data with process models to troubleshoot incidents and optimize operations even faster.
Learn how bp reduced crude purchasing decision time from two days to less than two hours across their refining operations.
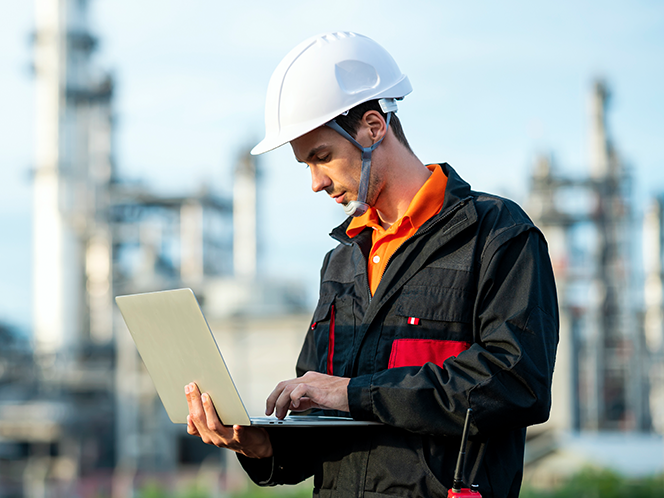
Related products
Redefine your processes to enable deeper collaboration, reduce value leaks and sustain productivity and innovation.
Use real-time and off-line process and economic data to maximize operating profit while satisfying regulatory requirements.
Achieve secure visibility into the supply chain with consistent data access across planning, scheduling, assay, and distribution.
Collect, aggregate and enrich real-time operations data for immediate problem-solving and easily deliver formatted data to enterprise applications and advanced analytics.
Empower your workforce—from engineers to business leaders
- Increase productivity with decision support and data availability, effective workflows and visualization capabilities.
- Attract, train, upskill and retain workers with experiential learning and institutionalized knowledge capture.
- Drive increased collaboration while adopting remote operations and new ways of working.
Learn how SLB integrated disparate data into a single visualization platform for agile, better-informed decision-making.
Related products
Capitalize on digital technologies and transform your business by integrating and visualizing all available data.
Build competence and confidence with a scalable solution that unifies experiential training tools in a cloud-based hub.
A self-service dashboarding tool for context-driven display, on-the-fly analysis, and secure sharing of your AVEVA™ PI System™ data—accessible anywhere on any device.
Visualize information in context with 3D, AR, MR and VR and collaboratively interact with your digital twin.